Work Package 4:
High-performance X-ray optics
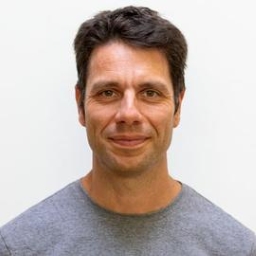
Q&A with Dr Maurizio Vannoni, HIR3X WP lead on “High-performance X-ray optics”
Q: Your group worked on optics contamination for the HIR3X project. How did this begin?
A: When the project started, we found ourselves quickly moving almost all meetings online due to the COVID lockdowns in Germany and the United States. Funnily enough I feel that this led to researchers from both countries speaking with each other more.
During one of those calls, we spoke about the problem of optics contamination in free electron lasers (FELs), where the beam intensities are so much higher than in synchrotrons. We often use mirrors to manipulate the FEL beam in certain ways to achieve the beam intensity and pulses required for an experiment. To put it simply, the radiation in these FELs is degrading the quality of the mirrors way faster than we are used to. This power, plus any human error that causes a mistake in the mirror’s alignment can cause serious damage to them.
These mirrors can also become “contaminated,” which can also happen in synchrotrons. Stray atoms can make their way into the chamber vessels of FELs and synchrotrons. When the high-energy beams interact with these atoms, they become very reactive and tend to stick to the mirrors. The mirrors then become less effective.
That’s a big problem, because these mirrors are crazy expensive. They need so much time to prepare that if you contaminate or destroy one of these mirrors, you must wait one year for a new one to be created and installed. The joke in optics is that the best way to maintain a mirror is to not have it in the first place!
How did you solve this?
In HIR3X, we wanted to improve mirror durability so that there is less down time for maintenance or waiting for a new mirror to be installed. In our research we found that mirrors coated in (B4C) boron carbide gives them double the damage threshold compared to not coating them. We already knew that boron carbide provided some protection, but not by how much. Getting a numerical measurement of its efficacy was new.
Surprisingly, in further experiments we also found that this coating exceeds this threshold in real-world experimental conditions; sometimes the coating can even offer five times the damage threshold for mirrors, compared to the calculations done in simulations. This is the fun part of being a researcher – often science replaces your original question with another one!
We can boost the accuracy of these simulations by considering that we are not dealing with electromagnetic waves, but rather photons. Simulations run in a more particle physics fashion gives a much more reasonable result that matches the experimental reality.
So overall we both understood more about the damage threshold of these mirror linings, and also how to run better simulations. These simulations are still not perfect, but they will give any researchers who want to use an FEL a more realistic idea of how their experiment will perform.
Why are these mirrors so important for FEL research?
The second aim we had in this project was to manipulate the FEL beam to provide more results for researchers when they scan their target material. The strategy we investigated was to create precise time delays between different photon colours in X-ray experiments.
In a free electron laser, the electrons are first accelerated and then the undulator guides the electrons to create photons. If you divide these undulators into two groups, one of these undulator groups can generate photons at one level of energy and the second group can generate photons at another energy level, which we call two “colours.” In this way the FEL can emit two photon energies at the same time, which means researchers can scan their target in two ways.
The problem with this setup is that the two photon energies are arriving at the target at the same time, which makes it difficult to scan. It would be much easier to scan the target by having one colour arrive first, followed by the second a short time later. We can do that by using magnets to send one group of electrons along a longer path before they enter the undulator. We call this longer path that delays the second colour a magnetic “chicane.”
How does research on chicanes help researchers get better data from FELs?
We can arrange things so that the second colour arrives to the target before the first. In this case we can delay colours by using a chicane of only mirrors. By using these two types of chicane for each colour, we can time both colours in any combination we like. For example, the first colour to arrive at the target can excite the material and then the second colour can take a picture of what happened femtoseconds later.
This is where things become interesting for researchers using chicanes. They can repeat this experiment, each time using the second colour to take a picture with slightly different delays. They can then combine these pictures to create a ‘movie’ of what happens during a certain chemical or physical process.
A fellow researcher described it to me in a great way. Imagine going to a football match and seeing two things: the players entering the stadium and then the final result. Where’s the fun in that? You didn’t see how the team won.
With these chicanes, researchers can see very fast chemical reactions or processes while they are happening.
You also worked with SLAC on many of these issues. What was the cooperation like?
Our collaboration was fantastic. We were able to visit each other’s sites, and although we didn't do experiments together, exchanging data between us helped us. For example, they have a metrology lab that can measure the degree of error of a mirror’s surface, and any contaminants on it. We were able to measure both our mirrors and compare results. Of course the results are always applied to slightly different systems. That said, seeing how similar technologies evolve in different research facilities helps to maintain a balance between scientific innovation and practical reliability. In other words, comparing our differences also helps to build realistic standards for our mirrors if they are used in other labs.
Speaking of the mirrors, at what stage are you now with them?
We are planning now to install these new damage-resistant mirrors in the second half of this year, and then next year will be the first commissioning. It’s exciting for me. You get to see how they behave in real conditions, and it's also big release when you finally see that the things are working well. You can give the results of your hard work to other colleagues, who can then use it for their real-world experiments.
Suggested research:
- Tavakkoly, Marziyeh, et al. "Simulations about stability, damage, and heating impacts for an x-ray optical delay line at sase3." Journal of Physics: Conference Series. Vol. 2380. No. 1. IOP Publishing, 2022.
- Serkez, Svitozar, et al. "Opportunities for two-color experiments in the soft X-ray regime at the European XFEL." Applied Sciences 10.8 (2020): 2728.
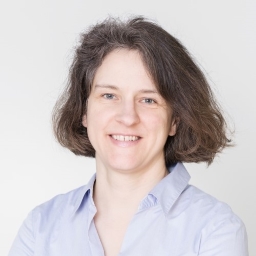
Q&A with Dr Elke Plönjes-Palm, HIR3X WP co-lead on “High-performance X-ray optics”
Q: What type of research does your working group do during HIR3X?
A: Broadly speaking, our work focuses on the development of the transport of the X-ray free electron laser (FELs) beams from the source to the experiment; it is a very long term endeavour of continuous improvement which, thus far, never really stops.
For this we need the best mirrors one can buy and we need to measure their quality and their performance to give feedback to our users as well as our supplier companies. Furthermore, at all FEL facilities, the optics groups develop new devices which allow shaping of the light beam. Two examples are so-called monochromators, which allow us to use only a small bandwidth of the light pulse for specific experiments mainly on solids and surfaces, for example catalysts or solar cells, and split-and-delay lines, which split the light pulse into two halves for time-resolved experiments.
First off, we really want to optimise the optics so that you get the cleanest and smallest possible focus. What you see at these short wavelengths is that any small roughness on a mirror’s surface can greatly limit the quality of your results. With the x-rays being so short, you basically see every little bump on the surface of the mirror.
We can also see this when we do simulations with an idealised, perfectly-smooth mirror surface. You get a perfect focus. Then, when you put in the data of the surface measurement of the real mirrors that you have installed, you can see how the real focus is different from the ‘perfect’ simulation. That's due to the reality of the optics.
What’s the second goal?
To judge the performance of the optics and the achieved focus it is important to develop diagnostics which measure the focus at the experimental station. One very convenient type are ‘wavefront sensing’ devices. We exchange information on the performance of such devices from the different facilities to see if they get the same results.
Once the beamline scientists and users have that, they can optimise the optics performance. Beyond the project our final, long-term goal is to develop an automated system that allows the user to tell the software “readjust from one micron to 10 microns” and then using wavefront sensing, it basically sets it to the right settings for the user.
The ultimate goal is to remove the pressure off the beamline scientists who otherwise need to be there practically 24 hours a day to make changes according to user requirements.
How did DESY, European XFEL and SLAC work in this project?
We at FLASH, European XFEL, and LCLS organised regular meetings where we discussed specific topics on optics. I think it was a really big benefit. The whole project started during the COVID-19 lockdowns, so we couldn't travel to meet each other, and we started to work online.
Once every month, or every other month, we discussed what was happening at the other facilities. Then, we chose a topic for the next meeting which we could discuss in a bit more depth. We all worked on our topics, and then we exchanged and got feedback and ideas on what maybe could be done better.
Sometimes we invited additional experts from the facilities to attend meetings where we discussed a specific topic. We ended up with a nice discussion platform of about six to 15 people, depending on the topic.
We just openly discussed any problems we had. I think this is a very beneficial approach, because at conferences you typically only talk about the successes. In the past, when video conferences were not yet common, typically we met once or twice a year to discuss our highlights.
It sounds like this openness was valued among everyone.
I think it’s really important to also discuss mistakes, because those are very rarely discussed in more formal settings. There’s a joke among scientists that it would be useful to have a “journal of failed experiments.” Usually when something fails, you don't present it in-depth at conferences because it's not publishable. Then the next facility tries something similar, only to once again fail.
Through these more informal and regular meetings, you can say early on that you are thinking of a certain approach to a problem, and another team can say “we already tried that and it doesn't work, perhaps try this.” I think this is one of the big benefits of such a collaboration that you do not get with just reading publications or attending conferences. It keeps each side updated during the process.
Do you see any commercial potential to this research?
For me the greatest potential lies in the faster operation of experiments and making the FELs easier to use - for both the expert user to those only interested in their sample, not the experimental environment or technique. This is something that all our fields now need to move towards, similar to how synchrotron sources are operated.
Perhaps in the long run, if the stability of the machines can be significantly improved, there could also be a commercial/industrial user base in areas where it's really important to look also at the time structure of certain processes in materials, for example catalysts.